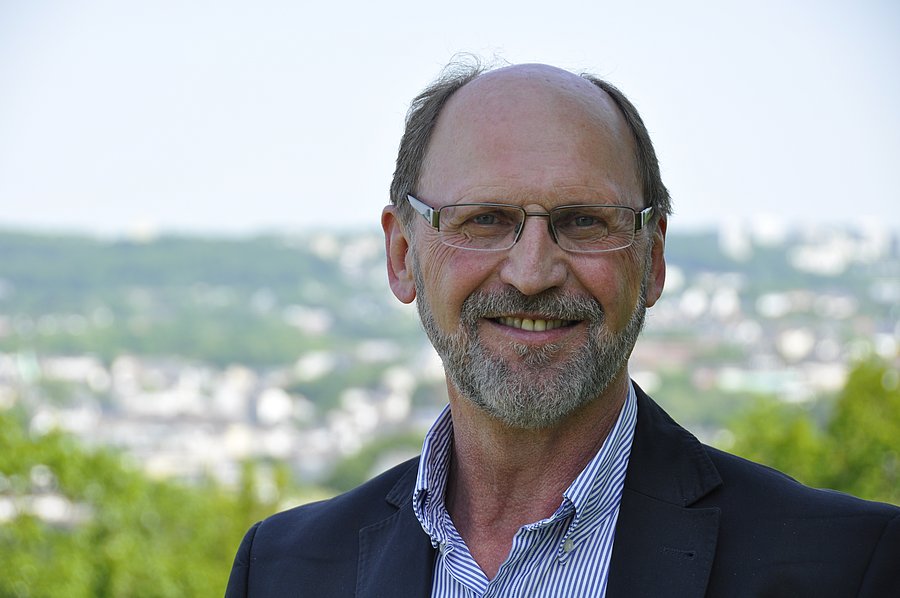
Transmutation - can nuclear waste be recycled?
Prof Dr Karl-Heinz Kampert / Astrophysics
Photo: UniService Transfer
Transmutation - can nuclear waste be recycled?
Wuppertal physicist Karl-Heinz Kampert on the possibilities of reprocessing nuclear waste
Nuclear power plants around the world produce a good 12,000 tonnes of nuclear waste every year; in Germany, around 1,600 tonnes have been produced over the years. The first Castor transport with spent nuclear fuel rods took place in 1995 to the Gorleben interim storage facility. To this day, politicians are still searching for a suitable final repository. However, radioactive waste can perhaps also be recycled, an idea that the nonprofessional can hardly imagine. The magic word in this context is 'transmutation'. Astrophysicist Professor Karl-Heinz Kampert from the University of Wuppertal knows how such a process works and what needs to be considered.
Transmutation
The first question to be answered in this context is where all this nuclear waste comes from. Kampert explains: "When we operate nuclear reactors or have operated them in the past, so-called nuclear burn-up occurs, i.e. we split uranium nuclei and then fission products are produced. These are radioactive and decay over a long period. At the same time, we also produce new fissile nuclei, such as plutonium. In our light water reactors, we not only burn plutonium, but we also produce plutonium. Moreover, that now adds up to many tonnes. These components, i.e. plutonium, but also other transuranium elements and fission products that are produced, are radioactive waste, and they have to be disposed of." So far, this radioactive waste has mainly been stored underground, where it remains highly active for several hundred thousand years. Nobody knows yet whether our storage sites will work for that long. At this point, the topic of transmutation comes to the fore. "There is the idea of actually changing this burn-up again, i.e. converting the long-lived isotopes, some of which have a half-life of many hundreds of thousands of years, into short-lived isotopes. Then the nuclear waste does not have to be carefully stored for many hundreds of thousands of years, but only over a time scale of one hundred and fifty or two hundred years. Transmutation is the mutation of nuclei into others that are shorter-lived."
No conversion without partitioning
The process of transmutation has been used indirectly in physics since the 1960s. "If you produce fuel for nuclear reactors, you have to have uranium 235 in sufficient concentration as fuel for conventional reactors. This occurs naturally at 0.7% in uranium mines, but reactors require 3-5% enrichment. The main isotope is uranium 238, which is virtually the natural uranium. There are so-called enrichment plants for enriching uranium 235." Plants of this type are a prerequisite for the process of transmutation, which, however, must always be mentioned in the same breath as the term partitioning. "So first I take the nuclear waste, separate it and extract certain isotopes, which I then transmute. However, this is extremely time-consuming and expensive. The upstream process of partitioning in particular is the more complex industry, because I have to do a lot of 'dirty chemistry' to separate these long-lived isotopes from the rest of the spent fuel." This is always referred to as P&T and only works in several cycles.
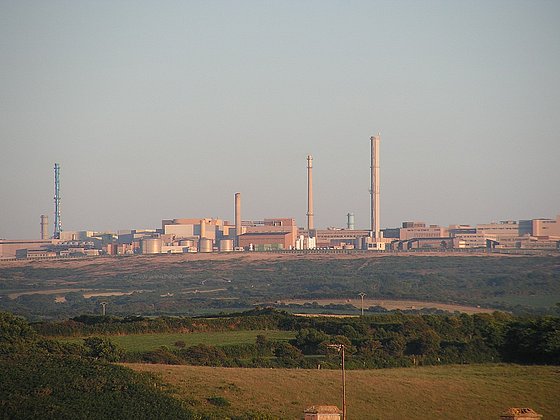
La Hague reprocessing plant (2005) CC BY-S 2.5
Reprocessing plants
Scientists now have the idea of simply converting the highly radioactive transuranium elements into other elements that radiate for less time and are less toxic in order to take the horror out of nuclear waste. "In principle, this is possible," Kampert confirms. "It is actually possible to convert the particularly long-lived isotopes, but it is extremely complex and expensive." As examples that we know from the press, he cites the reprocessing plants 'La Hague' in France and 'Sellafield' in England, which operated this 'dirty chemistry' and from which substances were always released into the environment. "We would then need a whole series of these plants, which would be much more complex than what we have done so far. This partitioning is particularly difficult and very controversial from an environmental point of view. Theoretically, it is possible, but it still needs to be developed for large-scale industrial application. We're not there yet."
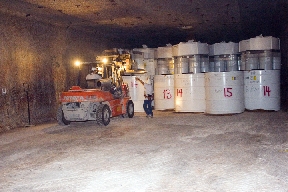
Transuranic waste in the Waste Isolation Pilot Plan, New Mexico, public domain
Should we recycle nuclear waste?
Germany has to store around 10,000 tonnes of nuclear waste. This corresponds to a volume of 20,000 cubic metres - four football pitches covered one metre high with nuclear waste. In addition to uranium, it also contains more than 100 tonnes of the highly radioactive and extremely toxic element plutonium. So could the transmutation process also be used to recycle nuclear waste? "Yes," answers Kampert spontaneously, "nuclear waste could indeed be recycled. If I think as a physicist, the burn-up is also valuable to a certain extent, because there is still a lot of uranium and plutonium in it. A type of reactor in Hamm-Uentrop and Kalkar never actually went into operation in Germany. Hamm-Uentrop started up briefly and Kalkar didn't. These are the so-called 'fast breeders'. Breeder means that they incubate their own fuel, and fast means that they work with fast neutrons." In simple terms, the principle is that you take natural uranium and change it a little. Natural uranium always consists of uranium 238 and a little plutonium. "If I now capture neutrons from uranium 238, then uranium 239 is produced and after a half-life of two days, i.e. quite quickly, plutonium 239. This means that every reactor also breeds plutonium. The fast breeder reactors are designed to take natural uranium and produce their own fuel, the plutonium. This is incredibly attractive from a physical point of view." There is a much higher utilisation of uranium because all uranium is used to breed plutonium. The plutonium is then burnt and recycled repeatedly. In conventional reactors, uranium 235 is always enriched to 3-5% by partitioning, and then incinerated and the waste is enriched again. In fast breeder reactors, uranium 238 is important because it is used to produce plutonium fuel in the reactor, which is then split to release the energy. This is of course attractive in terms of energy, but on the other hand, cooling is very expensive.
Transmutation by bombardment
A process known as partitioning and transmutation (P&T) already exists, at least on paper. Faster neutrons are used for this. By firing neutrons, for example, plutonium-239 with a half-life of 24,000 years could be converted into the stable ruthenium 104 and the short-lived caesium-134. "The idea is that I'm essentially transmuting all long-lived isotopes into shorter-lived isotopes." There are two approaches to transmutation. One is to use slightly modified nuclear reactors that are not optimised for energy production with the aim of converting as many isotopes as possible. This is already being pursued in various countries. "In Europe, there is another method that is technically very interesting: accelerator-based transmutation. Instead of using the reactor as a neutron source, a particle accelerator is used to generate neutrons. These are spallation neutron sources (in neutron production by spallation, six times less energy is used per neutron than in nuclear fission, editor's note). I shoot atomic nuclei at each other, but no long-lived isotopes are produced, instead neutrons come out. I use a powerful current accelerator, which I shoot at a target, generate neutrons and then have a neutron source. Furthermore, I can use this neutron source in a targeted way to convert certain isotopes." This is very controlled because if you switch off the accelerator, it is immediately over. This is the difference to a reactor, which always has long lead and lag times. "The accelerator-based technology is more elegant. It's as if you were working very specifically with a dissecting knife. The technology is there. But even then, I can't avoid doing the partitioning first, which is always necessary."
Transmutation - an alternative to permanent disposal storage?
According to the latest press reports, the permanent disposal of our nuclear waste is likely to be postponed for decades. However, would the P&T process really be an alternative? "Personally, I am sceptical," says Kampert promptly, "even the recommendation of the International Atomic Energy Agency and an expert report commissioned by the Federal Office for the Safety of Nuclear Waste Management (BASE) two years ago does not go there, although further research into it is recommended. Three alternatives are discussed in this recommendation. Firstly, these accelerators, which would bring many advantages, but would have to be accompanied by complex, expensive and dirty partitioning. Secondly, there are two reactor-based alternatives and, in the case of chemical partitioning, there are two different chemical processes, both of which are dirty. All of this is only feasible if we allow for at least 30 years of research from now on." In addition, there would be many other problems, as many reprocessing plants would have to be built in order to be able to carry out partitioning there. However, nobody wants that on his or her doorstep. "Transmutation also produces waste, which is short-lived but increases in quantity. I'm not getting rid of the radioactivity, I'm just changing its longevity into a short-lived one. Caesium and strontium are also a problem; we remember Chernobyl in 1986. After the reactor disaster, nobody was supposed to eat lettuce from the fields because caesium and strontium were washed onto the fields by the rain, could enter our bodies through food and damage our organs and bones." It is clear that uranium and plutonium can be shielded better than other isotopes via alpha decay. "Transmutation produces an evil that has a short live but a higher intensity. The process is complex and politically difficult to realise."
40% of radioactive waste is already vitrified
Kampert worked in Karlsruhe for several years and explains: "Nuclear waste was vitrified at the Karlsruhe Research Centre, which, like Jülich, was founded as a reactor research centre under Franz-Joseph Strauß. We've all seen the pictures of oil containers lying underground, rusting and leaking their contents. The so-called vitrification technology was developed over 20 years ago. Using this technology the waste is placed compactly in a glass container. Glass does not corrode, but it is still stored above ground in Karlsruhe because somehow they don't want to dispose of it. It was intended for final disposal because it is much better than the drums and around 40% of the radioactive material has already been vitrified. Here, too, we have to think about what we do with the vitrified material, because these materials can no longer be removed from the glass in order to partition them. The technology required for this process simply doesn't exist yet. So we don't have a holistic solution."
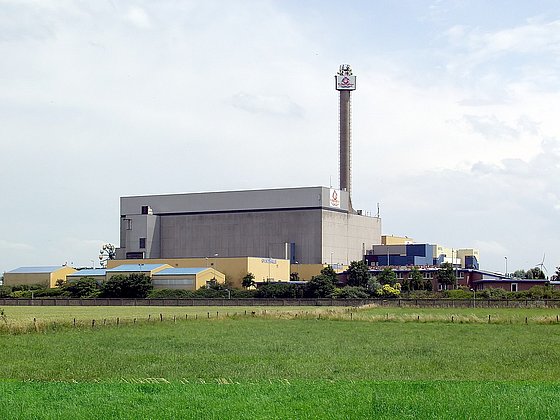
Schneller Brüter Kalkar (2004), later converted into the Kernwasser-Wunderland leisure park, CC BY-SA 4.0
Transmutation not socially accepted in Germany
In Germany, transmutation is not politically feasible and cannot be explained to the population, as they have already said goodbye to nuclear power plants, Kampert assures us, as they would have to invest in the unpopular technology again in order to transmute the burn-up. Other countries are taking a more progressive approach. France, for example, is even an international leader in processing and partitioning. In the Belgian city Mol, the first MYRRHA demonstration plant is even due to be launched in the 2030s. "The first prototype of an accelerator-driven plant in combination with a subcritical reactor will actually be built in Belgium. Construction of the €1.6 billion plant began in July this year. The Belgians have done a lot more nuclear power than we have and of course have the problem of waste disposal, but they have taken care of it. In addition, unlike in Germany, there was social acceptance there. You could certainly invest more, but the question is whether you're not casting out the devil with the Beelzebub, because the chemistry of the partitioning plants produces a lot of waste and other radioactive waste that also needs to be secured." The hope, of course, says Kampert in conclusion, is that the countries that are researching this might develop something that also produces something useful from the waste. Perhaps an industry will even emerge there that utilises the burn-off from other countries. In Germany, the population is currently lacking acceptance.
Uwe Blass
Prof Dr Karl-Heinz Kampert studied physics at the University of Münster from 1977 to 1983. From 1983 to 1986, Kampert was a research assistant at the University of Münster and received his doctorate in 1986. He then worked for three years as a postdoctoral research fellow at the large-scale research centre CERN in Switzerland. From 1989 to 1995, he was an assistant professor of physics at the University of Münster, during which time he qualified as a professor in 1993. He then taught as a professor of physics at the University of Karlsruhe and the Karlsruhe Research Centre, which both merged to form the Karlsruhe Institute of Technology in 2009. He has been teaching experimental physics at the University of Wuppertal since 2003.